Cracking the case of Arctic sea ice breakup
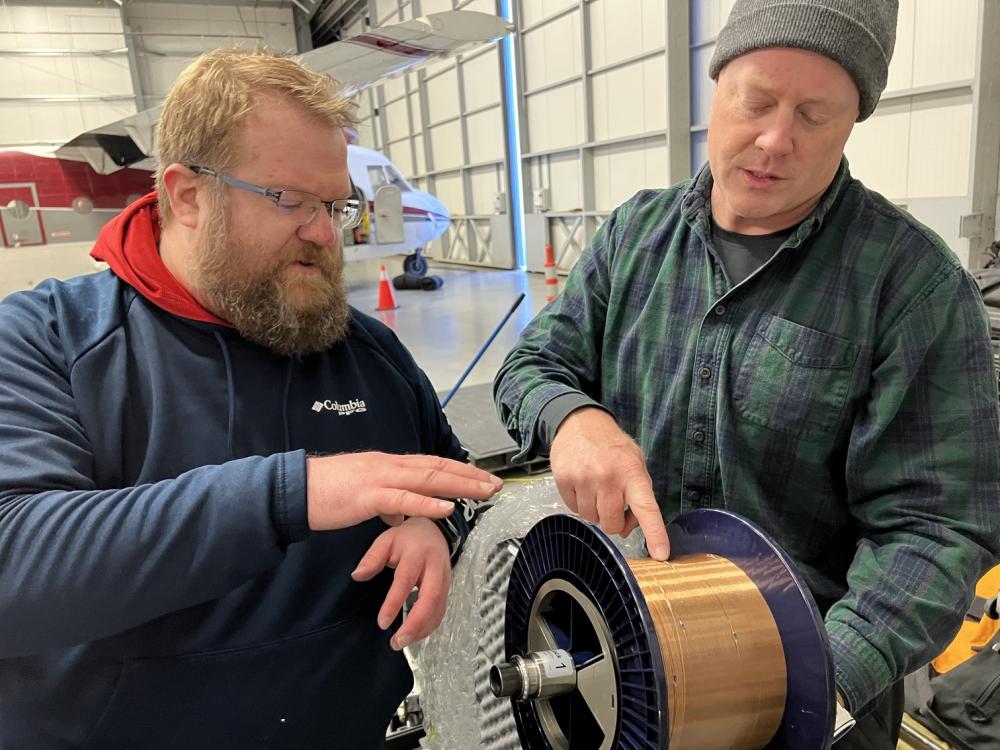
Despite its below-freezing temperatures, the Arctic is warming twice as fast as the rest of the planet. As Arctic sea ice melts, fewer bright surfaces are available to reflect sunlight back into space. When fractures open in the ice cover, the water underneath gets exposed. Dark, ice-free water absorbs the sun’s energy, heating the ocean and driving further melting — a vicious cycle. This warming in turn melts glacial ice, contributing to rising sea levels.
Warming climate and rising sea levels endanger the nearly 40 percent of the U.S. population living in coastal areas, the billions of people who depend on the ocean for food and their livelihoods, and species such as polar bears and Artic foxes. Reduced ice coverage is also making the once-impassable region more accessible, opening up new shipping lanes and ports. Interest in using these emerging trans-Arctic routes for product transit, extraction of natural resources (e.g., oil and gas), and military activity is turning an area traditionally marked by low tension and cooperation into one of global geopolitical competition.
As the Arctic opens up, predicting when and where the sea ice will fracture becomes increasingly important in strategic decision-making. However, huge gaps exist in our understanding of the physical processes contributing to ice breakup. Researchers at MIT Lincoln Laboratory seek to help close these gaps by turning a data-sparse environment into a data-rich one. They envision deploying a distributed set of unattended sensors across the Arctic that will persistently detect and geolocate ice fracturing events. Concurrently, the network will measure various environmental conditions, including water temperature and salinity, wind speed and direction, and ocean currents at different depths. By correlating these fracturing events and environmental conditions, they hope to discover meaningful insights about what is causing the sea ice to break up. Such insights could help predict the future state of Arctic sea ice to inform climate modeling, climate change planning, and policy decision-making at the highest levels.
"We’re trying to study the relationship between ice cracking, climate change, and heat flow in the ocean," says Andrew March, an assistant leader of Lincoln Laboratory's Advanced Undersea Systems and Technology Group. "Do cracks in the ice cause warm water to rise and more ice to melt? Do undersea currents and waves cause cracking? Does cracking cause undersea waves? These are the types of questions we aim to investigate."
Arctic access
In March 2022, Ben Evans and Dave Whelihan, both researchers in March’s group, traveled for 16 hours across three flights to Prudhoe Bay, located on the North Slope of Alaska. From there, they boarded a small specialized aircraft and flew another 90 minutes to a three-and-a-half-mile-long sheet of ice floating 160 nautical miles offshore in the Arctic Ocean. In the weeks before their arrival, the U.S. Navy's Arctic Submarine Laboratory had transformed this inhospitable ice floe into a temporary operating base called Ice Camp Queenfish, named after the first Sturgeon-class submarine to operate under the ice and the fourth to reach the North Pole. The ice camp featured a 2,500-foot-long runway, a command center, sleeping quarters to accommodate up to 60 personnel, a dining tent, and an extremely limited internet connection.
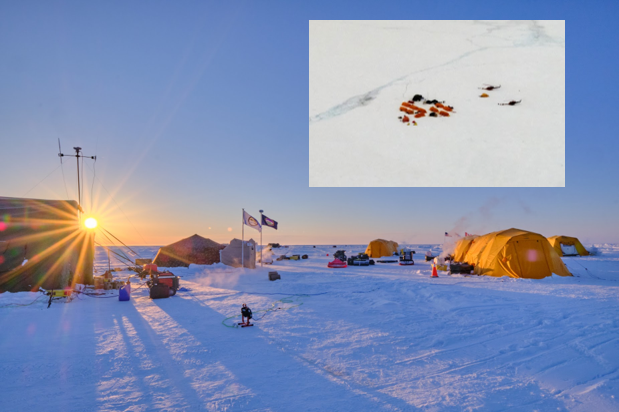
At Queenfish, for the next four days, Evans and Whelihan joined U.S. Navy, Army, Air Force, Marine Corps, and Coast Guard members, and members of the Royal Canadian Air Force and Navy and United Kingdom Royal Navy, who were participating in Ice Exercise (ICEX) 2022. Over the course of about three weeks, more than 200 personnel stationed at Queenfish, Prudhoe Bay, and aboard two U.S. Navy submarines participated in this biennial exercise. The goals of ICEX 2022 were to assess U.S. operational readiness in the Arctic; increase our country's experience in the region; advance our understanding of the Arctic environment; and continue building relationships with other services, allies, and partner organizations to ensure a free and peaceful Arctic. The infrastructure provided for ICEX concurrently enables scientists to conduct research in an environment — either in person or by sending their research equipment for exercise organizers to deploy on their behalf — that would be otherwise extremely difficult and expensive to access.
In the Arctic, windchill temperatures can plummet to as low as 60 degrees Fahrenheit below zero, cold enough to freeze exposed skin within minutes. Winds and ocean currents can drift the entire camp beyond the reach of nearby emergency rescue aircraft, and the ice can crack at any moment. To ensure the safety of participants, a team of Navy meteorological specialists continually monitors the ever-changing conditions. The original camp location for ICEX 2022 had to be evacuated and relocated after a massive crack formed in the ice, delaying Evans' and Whelihan's trip. Even the newly selected site had a large crack form behind the camp and another crack that necessitated moving a number of tents.
"Such cracking events are only going to increase as the climate warms, so it's more critical now than ever to understand the physical processes behind them," Whelihan says. "Such an understanding will require building technology that can persist in the environment despite these incredibly harsh conditions. So, it's a challenge not only from a scientific perspective but also an engineering one."

"The weather always gets a vote, dictating what you're able to do out here," adds Evans. "The Arctic Submarine Laboratory does a lot of work to construct the camp and make it a safe environment where researchers like us can come to do good science. ICEX is really the only opportunity we have to go onto the sea ice in a place this remote to collect data."
A legacy of sea ice experiments
Though this trip was Whelihan's and Evans' first to the Arctic region, staff from the Laboratory's Advanced Undersea Systems and Technology Group have been conducting experiments at ICEX since 2018. However, because of the Arctic's remote location and extreme conditions, data collection has rarely been continuous over long periods of time or widespread across large areas. The team now hopes to change that by building low-cost, expendable sensing platforms consisting of co-located devices that can be left unattended for automated, persistent, near-real-time monitoring.
"The Laboratory's extensive expertise in rapid prototyping, seismoacoustic signal processing, remote sensing, and oceanography make us a natural fit to build this sensor network," says Evans.

In the months leading up to the Arctic trip, the team collected seismometer data at Firepond, part of the Laboratory’s Haystack Observatory site in Westford, Massachusetts. Through this local data collection, they aimed to gain a sense of what anthropogenic (human-induced) noise would look like so they could begin to anticipate the kinds of signatures they might see in the Arctic. They also collected ice melting/fracturing data during a thaw cycle and correlated these data with the weather conditions (air temperature, humidity, and pressure). Through this analysis, they detected an increase in seismic signals as the temperature rose above 32-degrees Fahrenheit – an indication that air temperature and ice cracking may be related.
A sensing network
At ICEX, the team deployed various commercial-off-the-shelf sensors and new sensors developed by the Laboratory and University of New Hampshire (UNH) to assess their resiliency in the frigid environment and to collect an initial dataset.
"One aspect that differentiates these experiments from those of the past is that we concurrently collected seismo-acoustic data and environmental parameters," says Evans.
The commercial technologies were seismometers to detect the vibrational energy released when sea ice fractures or collides with other ice floes; a hydrophone (underwater microphone) array to record the acoustic energy created by ice-fracturing events; a sound speed profiler to measure the speed of sound through the water column; and a conductivity, temperature, and depth (CTD) profiler to measure the salinity (related to conductivity), temperature, and pressure (related to depth) throughout the water column. The speed of sound in the ocean primarily depends on these three quantities.
To precisely measure the temperature across the entire water column at one location, they deployed an array of transistor-based temperature sensors developed by the Laboratory's Advanced Materials and Microsystems Group in collaboration with the Advanced Functional Fabrics of America Manufacturing Innovation Institute. The small temperature sensors run along the length of a thread-like polymer fiber embedded with multiple conductors. This fiber platform, which can support a broad range of sensors, can be unspooled hundreds of feet below the water's surface to concurrently measure temperature or other water properties — the fiber deployed in the Arctic also contained accelerometers to measure depth — at many points in the water column. Traditionally, temperature profiling has required moving a device up and down through the water column.
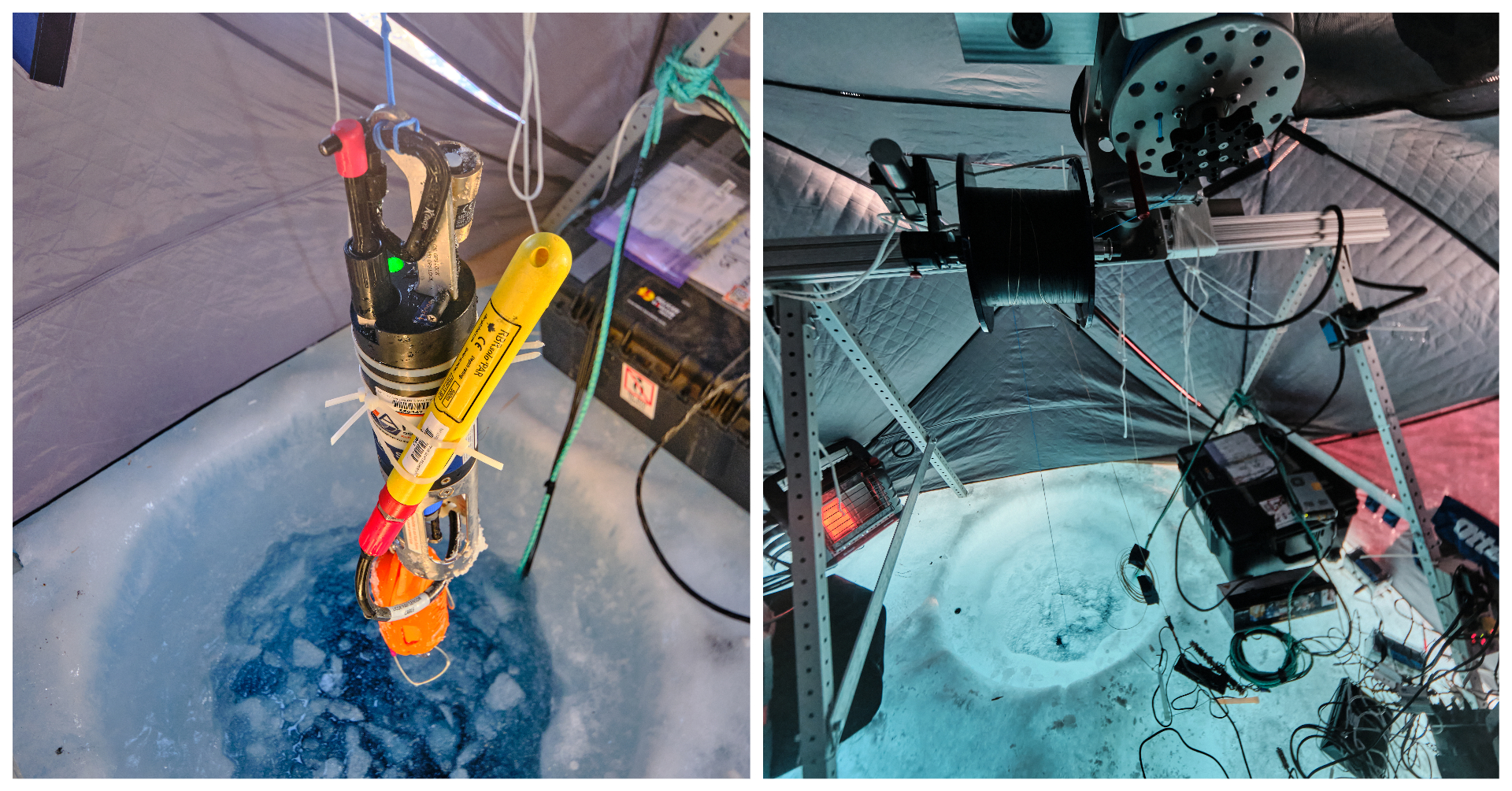
The team also deployed a high-frequency echosounder supplied by Anthony Lyons and Larry Mayer, collaborators at UNH’s Center for Coastal and Ocean Mapping. This active sonar uses acoustic energy to detect internal waves, or waves occurring beneath the ocean’s surface.
"You may think of the ocean as a homogenous body of water, but it's not," Evans explains. "Different currents can exist as you go down in depth, much like how you can get different winds when you go up in altitude. The UNH echosounder allows us to see the different currents in the water column, as well as ice roughness when we turn the sensor to look upward."
"The reason we care about currents is that we believe they will tell us something about how warmer water from the Atlantic Ocean is coming into contact with sea ice," adds Whelihan. "Not only is that water melting ice but it also has lower salt content, resulting in oceanic layers and affecting how long ice lasts and where it lasts."
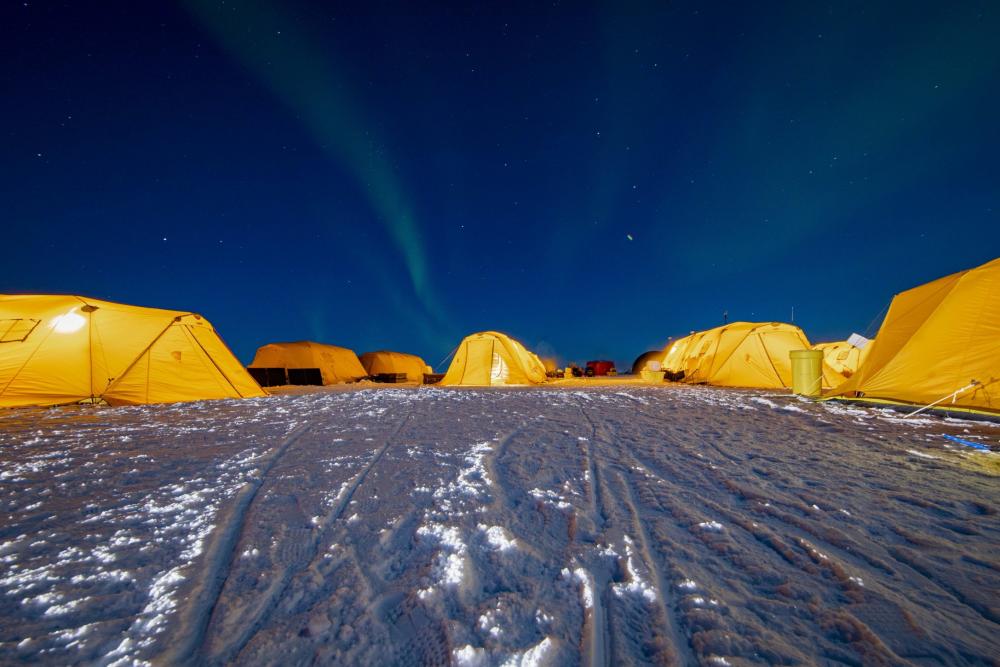
Back home, the team has begun analyzing their data. For the seismic data, this analysis involves distinguishing any ice events from various sources of anthropogenic noise, including generators, snowmobiles, footsteps, and aircraft. Similarly, the researchers know their hydrophone array acoustic data are contaminated by energy from a sound source that another research team participating in ICEX placed in the water. Based on their physics, icequakes — the seismic events that occur when ice cracks — have characteristic signatures that can be used to identify them. One approach is to manually find an icequake and use that signature as a guide for finding other icequakes in the dataset.
From their water column profiling sensors, they identified an interesting evolution in the sound speed profile 30 to 40 meters below the ocean surface, related to a mass of colder water moving in later in the day. The group's physical oceanographer believes this change in the profile is due to water coming up from the Bering Sea, water that initially comes from the Atlantic Ocean. The UNH-supplied echosounder also generated an interesting signal at a similar depth.
"Our supposition is that this result has something to do with the large sound speed variation we detected, either directly because of reflections off that layer or because of plankton, which tend to rise on top of that layer," explains Evans.
A future predictive capability
Going forward, the team will continue mining their collected data and use these data to begin building algorithms capable of automatically detecting and localizing — and ultimately predicting — ice events correlated with changes in environmental conditions. To complement their experimental data, they have initiated conversations with organizations that model the physical behavior of sea ice, including the National Oceanic and Atmospheric Administration and the National Ice Center. Merging the Laboratory’s expertise in sensor design and signal processing with their expertise in ice physics would provide a more complete understanding of how the Arctic is changing.
The Laboratory team will also start exploring cost-effective engineering approaches for integrating the sensors into packages hardened for deployment in the harsh environment of the Arctic.
"Until these sensors are truly unattended, the human factor of usability is front and center," says Whelihan. "Because it's so cold, equipment can break accidentally. For example, at ICEX 2022, our waterproof enclosure for the seismometers survived, but the enclosure for its power supply, which was made out of a cheaper plastic, shattered in my hand when I went to pick it up."
The sensor packages will not only need to withstand the frigid environment but also be able to "phone home" over some sort of satellite data link and sustain their power. The team plans to investigate whether waste heat from processing can keep the instruments warm and how energy could be harvested from the Arctic environment.
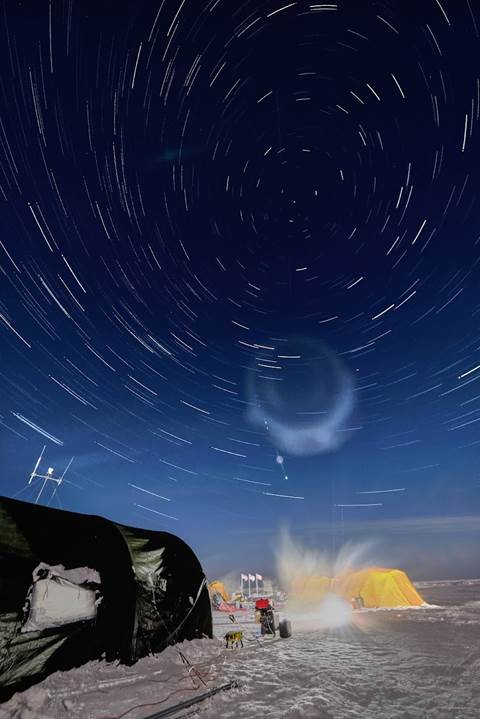
Before the next ICEX scheduled for 2024, they hope to perform preliminary testing of their sensor packages and concepts in Arctic-like environments. While attending ICEX 2022, they engaged with several other attendees — including the U.S. Navy, Arctic Submarine Laboratory, National Ice Center, and University of Alaska Fairbanks (UAF) — and identified cold room experimentation as one area of potential collaboration. Testing can also be performed at outdoor locations a bit closer to home and more easily accessible, such as the Great Lakes in Michigan and a UAF-maintained site in Utqiagvik (formerly named Barrow), Alaska. In the future, the Laboratory team may have an opportunity to accompany U.S. Coast Guard personnel on ice-breaking vessels traveling from Alaska to Greenland. The team is also thinking about possible venues for collecting data far removed from human noise sources.
"Since I've told colleagues, friends, and family I was going to the Arctic, I've had a lot of interesting conversations about climate change and what we're doing there and why we're doing it," Whelihan says. "People don't have an intrinsic, automatic understanding of this environment and its impact because it's so far removed from us. But the Arctic plays a crucial role in helping to keep the global climate in balance, so it's imperative we understand the processes leading to sea ice fractures."
This work is funded through Lincoln Laboratory's internally administered R&D portfolio on climate.
Inquiries: contact Ariana Tantillo.
Related Links
- U.S. Navy press release: "Navy Supports Scientific Research at ICEX 2022"
- ABC News video clip with Lincoln Laboratory researchers interviewed: "US, Russia vie for superiority in Arctic Circle"